Preclinical models of cerebral haemorrhage
Haemorrhagic strokes account for 10-15% of all strokes. In 40% of patients, haemorrhagic strokes are fatal within one month, rising to 54% after 1 year. Haemorrhagic strokes have a devastating effect on the quality of life of surviving patients, with only 12 to 39% able to get back to long-term independence.
Rapid diagnosis, stopping the haemorrhage and draining the haematoma, initiating treatment against blood poisoning, limiting vasospasms, neuroprotection and finally stimulating functional recovery are all possible strategies for reducing the number of deaths, promoting long term recovery and improving patients’ quality of life. Unfortunately, few pharmacological solutions are available.
The entire medicines R&D sector, from bench to bedside, is rising to the challenge of developing new, effective therapies. From initial proof of concept, through preclinical development, the use of appropriate tools maximises the chances of a successful translation. Whether the target is subarachnoid haemorrhage (SAH), intercranial haemorrhages (ICH) or evaluating the risks of haemorrhagic transformation following the administration of thrombolytic therapy, the choice of animal model and experimental design are key.
Our R&D team, in partnership with that of Professor Denis Vivien (U1237, INSERM/UNICEAN, Caen) has developed several haemorrhagic stroke models using our insight into the issues involved. They are suitable for use in rodents and designed to respond to a wide range of questions surrounding the preclinical development of new therapies linked to cerebral haemorrhage. As in the clinical setting, we assess both the impact of haemorrhage on the brain tissue using functional magnetic resonance imaging (MRI) and functional recovery via measurement of behavioural parameters. We also offer tools which allow more precise investigation of the process of neuroinflammation, such as molecular imaging using MRI, flow cytometry, and various other immunohistochemistry markers (Neutrophiles and NETS, activated microglia, etc.).
Let’s take a more detailed look at each model!
ICH models
These models, which are well documented in the literature (see Goulay et al., 2017), have been enhanced by the STROK@LLIANCE R&D team.
The models are induced in rats and mice using intraparenchymal injections of collagenase (collagenase model), or of autologous blood (blood injection model) directly into the striatum. Collagenase model: following damage to the vascular wall collagen, the surrounding brain tissue becomes flooded with blood. In this model the extent of the haemorrhage is well controlled; the site of the injection determines whether or not the blood leak affects the lateral ventricles. Blood injection model: a fixed volume of autologous blood is injected. The main difference between the two models is that there is a real bleed in the collagenase model, whereas an artificial haematoma is created in the blood injection model. The collagenase model is more physiological, but the volume of bleeding can be influenced by the administration of the compound, which is not necessarily the aim of the study. Tools: in both models, we monitor development of the haemorrhage in rats and mice using MRI (T2 & T2* imaging), using molecular imaging of the inflammation (endothelial activation of VCAM-1), behavioural assessment and immunohistochemistry.
>> More information about ICH models
SAH models
SAH models unfortunately remain unsatisfactory at the moment in terms of their similarity to real clinical situations. Professor Vivien’s team published a literature review in November 2021 in Frontiers in cardiovascular disease, taking stock of the current situation and pointing out the models’ shortcomings:
>> link to article : Delayed Cerebral Ischaemia After Subarachnoid Haemorrhage: Is There a Relevant Experimental Model? A Systematic Review of Preclinical Literature (Goursaud et al., 2021). In this review the SAH model which uses the autologous blood injection in the cisterna magna, although not perfect, is described as the best alternative available for testing molecule effectiveness.
Haemorrhagic transformation or over bleeding model
In the development of molecules for the treatment of thromboembolic/ischemic stroke, the question of safe utilisation – particularly in relation to bleeding – is central for neurologists and assessment agencies. One key issue is to determine whether the risk of haemorrhagic transformation is increased with the use of the candidate drug. Another is whether the product increases the volume of bleeding (over bleeding) when haemorrhagic transformation occurs (the risk of transformation being present in all patients). The risk of over bleeding can be assessed using the collagenase model described above, to measure the effect a molecule of interest has on bleeding. >> More information about ICH models
Our R&D team is currently developing a new model in diabetic mice to meet the need for a reliable model for assessing the risk of haemorrhagic transformation. Watch this space in September 2022, to see the presentation of validation data.
ICH models: our results
The aim of the collagenase model is to mimic the effects of an intraparenchymal brain haemorrhage
This model is induced by the direct, unilateral injection of collagenase in the striatum. It results in damage to the adventitia (external layer of the vascular wall), which causes haemorrhaging around the injection site. By choosing the structure, concentration and volume of collagenase it is possible to obtain a reproducible haemorrhage associated with a brain lesion and well-defined motor defects.
MRI & molecular imaging
Changes in the volume of both the lesion (T2) and the haematoma (T2*) can be located, measured and tracked with MRI brain imaging. (Illustration: Figure 1: rat on day 1 , Figure 2: mouse on day 1).
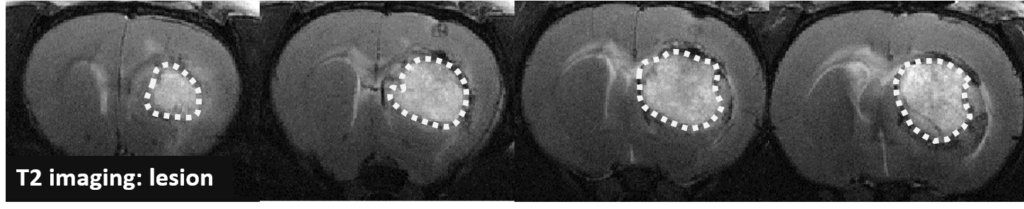
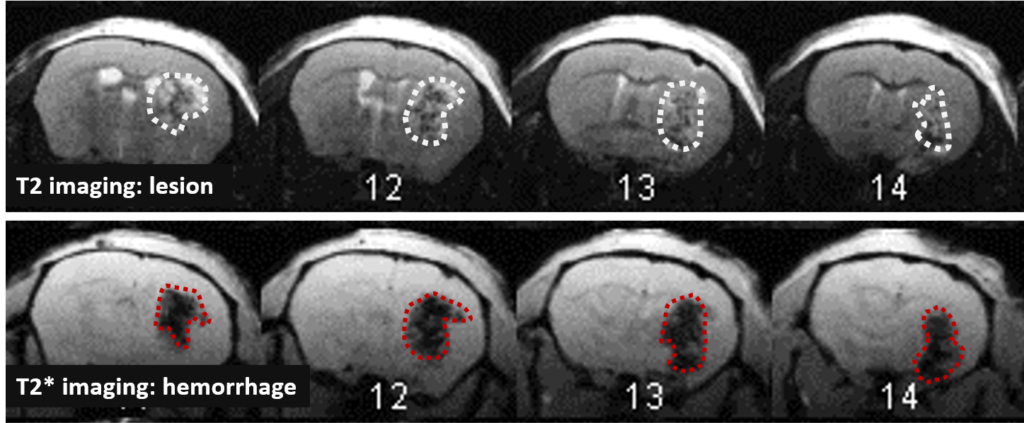
This type of measurement can answer questions relating to the efficacy of a treatment on haem toxicity, oxidative stress linked to the release of iron oxide, increase in intracranial pressure and the capacity of a treatment to reduce brain lesions through different processes.
Long term monitoring enables researchers to highlight the impact of a treatment over the longer term and to observe the animal’s functional recovery phase (Figure 3). It also makes it possible to track the reduction in post-induction oedema and tissue remodelling in the damaged area. The activation of those processes results in a reduction in the size of the lesion (phagocytosis of cellular debris, glial colonisation, etc.).
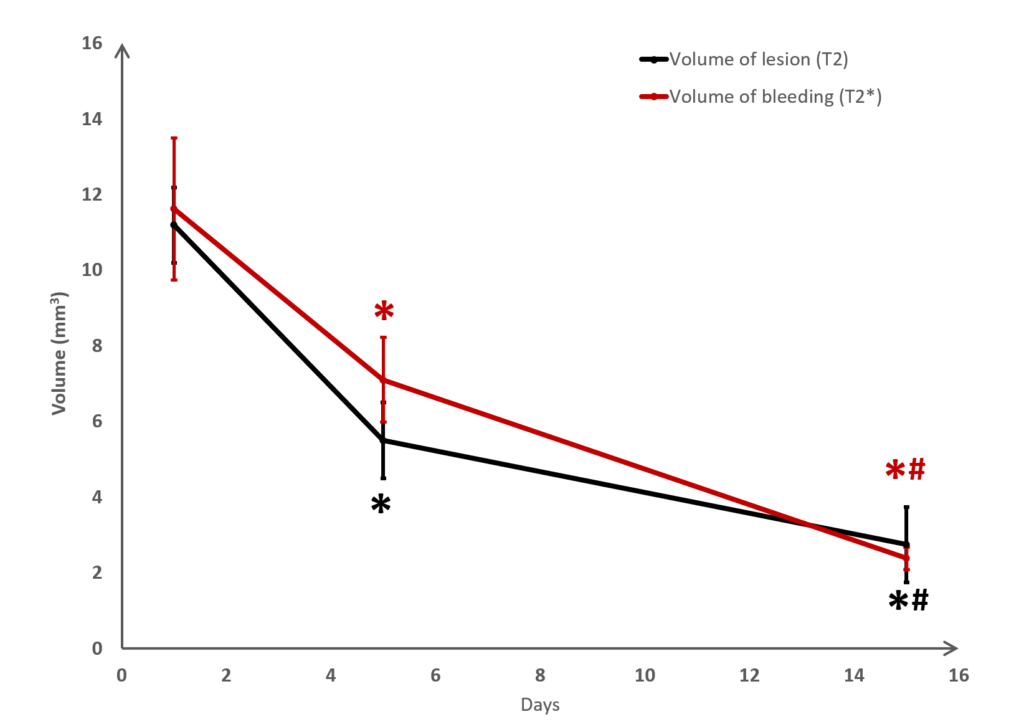
It is also possible to use this model to acquire data relating to inflammation. A protein of interest can be visualised in vivo via molecular imaging, using microparticles of iron oxide (MPIO) coupled with an antibody. By targeting markers such as VCAM-1 or P-Selectine it is possible to track and quantify the level of brain inflammation (Figure 4).
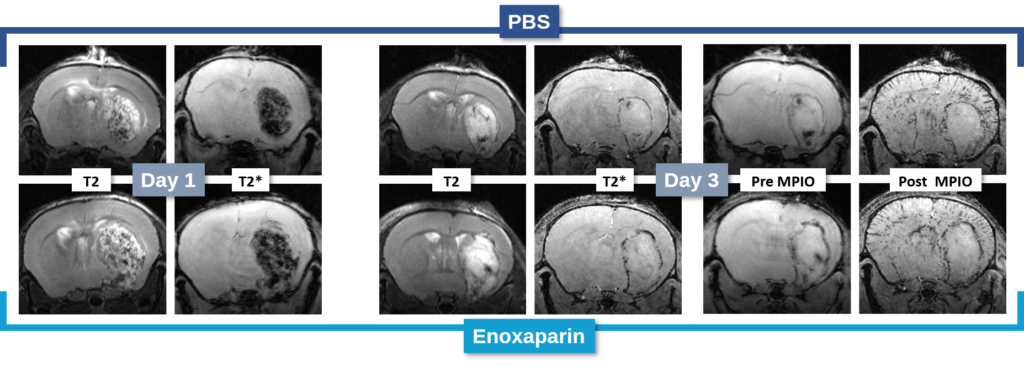
In the study of our ICH model, we assessed the impact of an anticoagulant agent (such as Enoxaparin). We observed that Enoxaparin produced an increase in the volume of the haemorrhage at day 1 (Figure 4) as well as an increase in the size of the lesion at day 3 (Figure 5). With this model it is possible to assess a susceptible molecule’s risk of bleeding to provoke over bleeding.
By using MPIOs coupled with a V-CAM1 antibody, we have been able to quantify in vivo the impact of Enoxaparin pre-treatment on the expression of this cell adhesion molecule and thus demonstrate the level of post-haemorrhage inflammation (Figures 4 and 5).
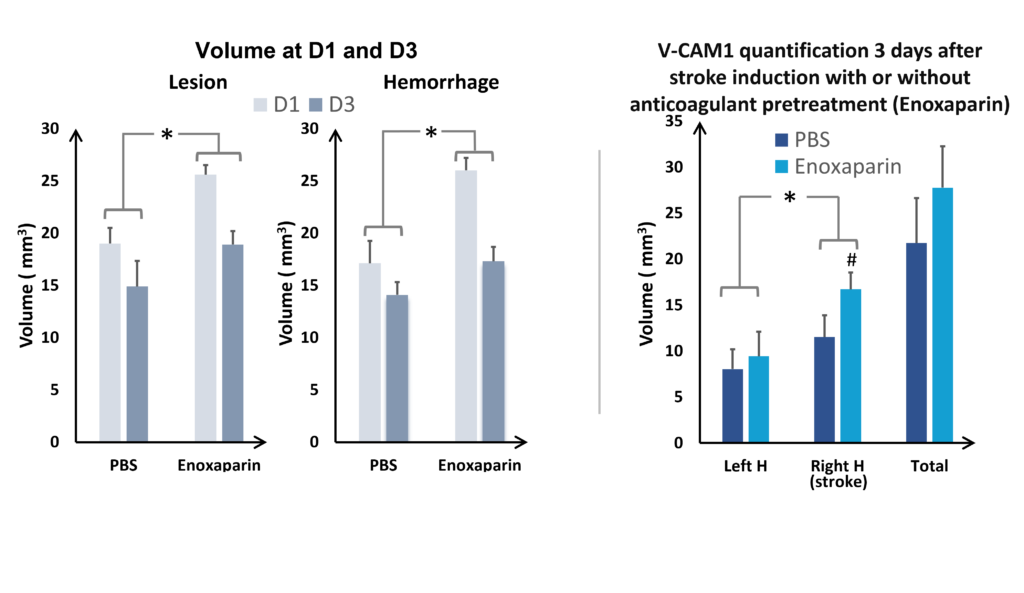
and VCAM quantification at D3 (on the right), hemispheres comparaison (ANOVA; *p<0.05; Mean +/- SEM); Effect of Enoxaparin VS PBS (ANOVA; *p<0.05) H= hemisphere
Immunohistochemistry labeling
This molecular imaging data can be supplemented by immunohistochemistry studies. Following tissue sampling, we can, for example, quantify:
- The activation of neutrophiles, macrophages/monocytes and microglial cells via an anti-MPO antibody
- The implementation of Neutrophil Extracellular Traps (NETs) via the Anti-H3Cit antibody
- The presence of neutrophiles via the Anti-Ly-6G antibody
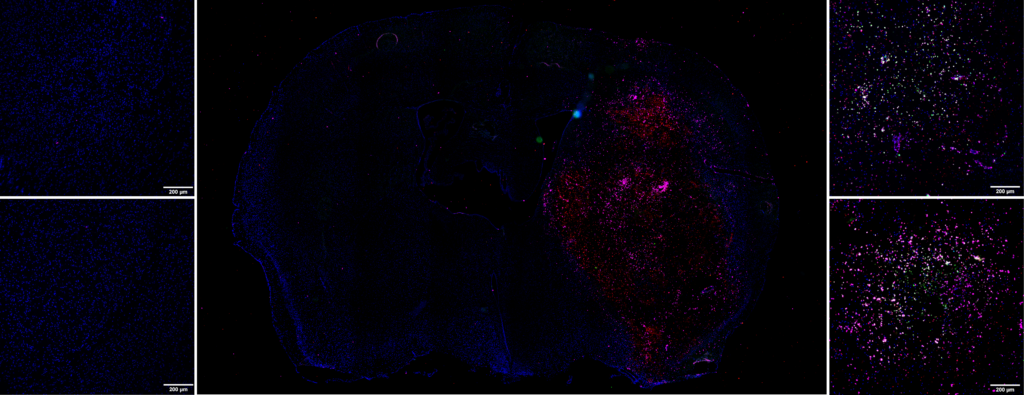
Functional assessments
Functional assessments also have an essential role in the translational aspect of this model. By monitoring the changes in the rotational biases and the motor deficits induced by the unilateral brain lesion in the corner test, it is possible to quantify the degree of deficits the animals have sustained. Illustrated in Figure 7 (Lon the left) in rats, rotation bias is particularly marked in animals having received collagenase: whereas the sham animals rotate equally to left and right, the brain-damaged animals rotate only to one side. Longitudinal monitoring enables us to observe the evolution of the deficits over the longer term, for example by measuring the strength of the back paws of mice (See figure 7 on the right). Here it is interesting to note the presence of hemiparesis, that is a lessening of the strength of the left paw (affected) compared to the right paw (healthy). Despite a certain degree of spontaneous recovery, the deficits are visible for at least three weeks after induction.
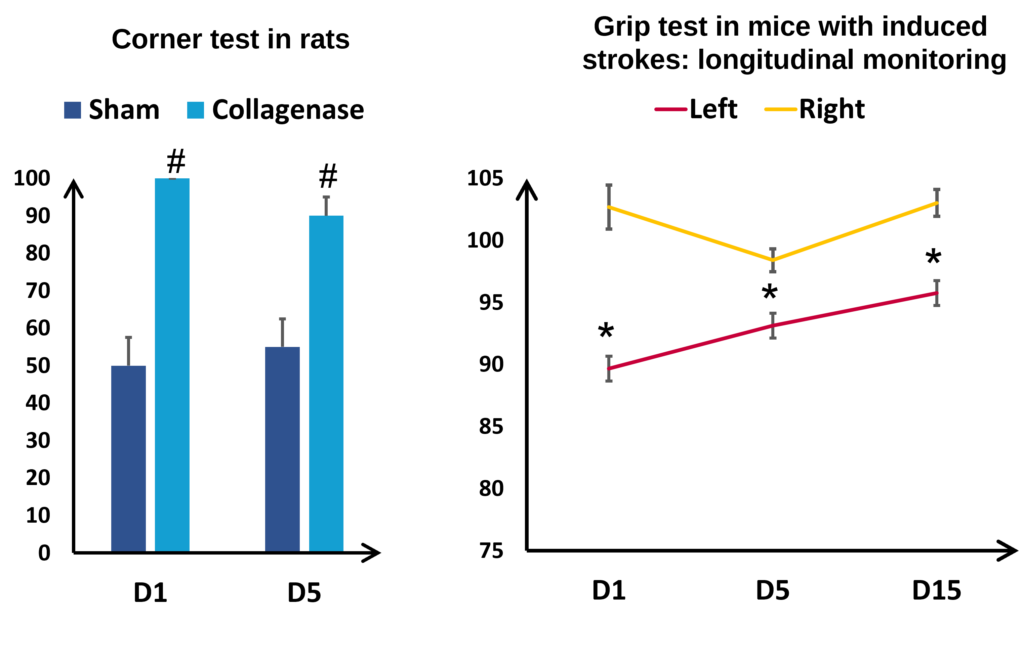
Taking this model a little further
It is also possible to use this model to assess the risks of increasing the volume of bleeding associated with a treatment. This is a central issue in the development of a neuroprotective drug for use in haemorrhagic and ischemic strokes and for anticoagulant treatments used in the prevention of thrombotic strokes. In the collagenase model, anticoagulants such as Warfarin and Enoxaparin clearly produce over bleeding. These treatments can be used as a reference point for assessing the risk linked to the use of new molecules (see Figures 4 and 5)
As regards the risk of haemorrhagic transformation, our R&D team is currently developing a new, more translational model. Watch this space in September 2022 for more information.
Thus, by combining the experience of our experts with the brain imaging data, behavioural results and histological analyses of this ICH model, Strok@lliance can provide you with answers to your questions around ICH and the preclinical development of new treatments.
Contact our experts if you’d like to know more!
The STROK@LLIANCE core mission is to support the development of new therapeutics.